Through-bond Energy Transfer Cassettes
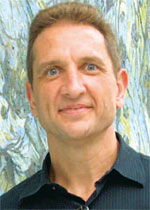 |
Aurore Loudet, Cliferson Thivierge, and Kevin Burgess*
Department of Chemistry,
Texas A & M University,
Box 30012, College Station, TX 77841, USA |
Through-bond Energy Transfer
What Is It?
Fluorescence resonance energy transfer (FRET) is one means by which energy can relay between fluorophores in close proximity. Underlying theories for FRET, as described by Förster, 1, 2) highlight its physical limitations: distance between the donor and acceptor is the most important, but others include orientation between the fluor transition dipoles, and the overlap integral. Overlap integral represents the intersection of the donor fluorescence with the acceptor absorbance; 1,3) poor overlap gives inefficient energy transfer (Figure 1).4)
Unlike FRET, energy transfer can occur through bonds in which a donor relays energy to an acceptor predominantly via twisted π-electron systems. These have been investigated in materials research 5, 6) and for model photosynthetic systems; † 9-11) while our group was the first to form fluorescent labels for biotechnological applications 12) using this concept. 13-20) Theories describing through-bond energy transfer (TBET) are vague; indeed, TBET may really be a summation of several means of transferring energy. These mechanisms may operate simultaneously with FRET, but are usually much faster.
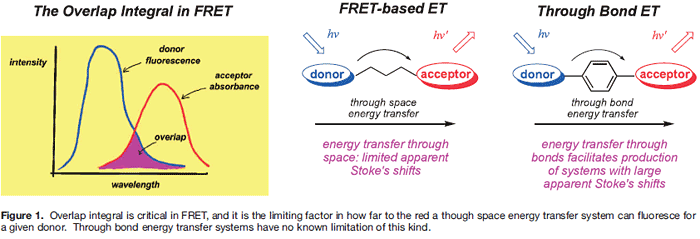
† Through bond energy transfer effects have been studied in detail for long conjugated polymers.7)
Studies indicate that emissions tend to occur from the lowest energy state in the polymer conformation. Related to this, a single “defect” in the polymer chain can cause the whole system to photobleach in a single step, ie the system does not behave as if it was a series of dyes in a chain isolated by twists in the conformation.8)
▲ページのトップへ
There are at least two key differences between through-space and through-bond energy transfer. First, there is no known constraint in TBET that corresponds to the overlap integral. Secondly, the donor and acceptor parts are more intimately connected in TBET systems than in ones where there is no
possibility of π-orbital overlap between them. For instance, the
behavior of each fluor entity might be more sensitive to REDOX
events than it is in FRET systems.21-24)
There are several implications of the discussion above in the applications of TBET in biotechnology. First, separation of excitation and observation wavelengths is potentially useful where large dispersion between the donor absorbance and acceptor fluorescence facilitates visualization of the dye.
Second, the “greater intimacy” of the donor and acceptor pairs relative to FRET means there are more possibilities to switch the TBET between on and off states. This is potentially useful since even if TBET is not occurring, the cassettes may still fluoresce from the donor part, ie they are always on. Finally, if the donor fluor is sensitive to photobleaching, it may be less so in the cassette. Figure 2 outlines the reasons for this in the example of a fluorescein-like donor and a rhodamine-like acceptor.
Fluoresceins are unstable to photobleaching, mainly because their excited states undergo rapid singlet-to-triplet crossover, and the triplet states are longer-lived and reactive towards oxygen. If donor-to-acceptor energy transfer in a TBET cassette is faster than the crossover process, then the energy is preferentially relayed to the rhodamine part. Rhodamines tend to fluoresce quicker than they undergo singlet-to-triplet crossover, hence the photostability of the cassette overall is better than the fluorescein donor; it is more like that of the rhodamine acceptor.
▲ページのトップへ
Design of TBET Systems For Biotechnology
When preparing TBET cassettes, the following parameters are critical:
● donor with high extinction coefficients at a relevant excitation wavelength
(eg corresponding to a relevant laser source);
● a twist between the donor π-system and that of the acceptor; and,
● acceptors that fluoresce with high quantum yields and sharp emissions at appropriate wavelengths.
Outside of materials research, the Lindsey group were one of the first to prepare through-bond energy transfer cassettes that absorb above ca 488 nm, one example being the porphyrin derivative 1. Lindsey was interested in compounds like this as models for photosynthesis.25) Our work on preparing smaller systems intended for biotechnology 12) began with some lipophilic cassettes like 2 and the surprisingly insoluble compound 3. 14, 15, 17) Other groups, notably Akkaya 26-29) and Ziessel 30 ,31) have since made other lipophillic TBET cassettes, mainly to examin etheir luorescence properties.
We defined a parameter we call “energy transfer efficiencies” (ETEs) as { Фd /Фa } x 100 32) where Фd is the quantum yield of the cassette when excited atthe donor, and Фa is the same parameter except when the cassette is excited at the acceptor.
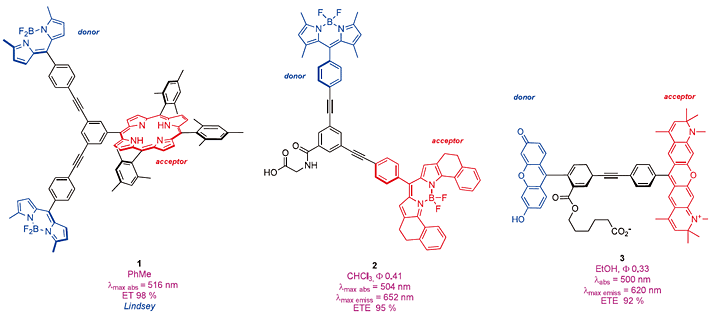
This reflects the efficiency with which the cassette transfers energy from the donor to the acceptor. The brightness of a TBET cassette depends on the extinction coefficient of the donor part(s), the efficiency of the energy transfer, and the quantum yield of the acceptor fluor in the cassette.
Unfortunately, dyes like 1 - 3 are insoluble in aqueous media.
Cassettes 2 and 3 have functional groups to allow attachment to biomolecules, and 3 has the type of structure that looks like it might have water solubility, but it does not. In general, it is far more difficult to prepare water-soluble TBET cassettes 18, 33) than lipophilic ones. A breakthrough for our research came when we realized a practical method to sulfonate BODIPY dyes.34) Eventually, this facilitated the syntheses of a series of TBET cassettes including
4 and 5. 32) These are sufficiently fluorescent on proteins in aqueous media to be used in multiplexing experiments in which the dyes are excited at about 500 nm, and fluoresce at 600 or 650 nm respectively.
▲ページのトップへ
Application of TBET Cassettes In Observing Protein-protein Interactions
Our concept of multiplexing with TBET cassettes is outlined in Figure 3. The hypothetical purpose of this experiment is to determine which of several (here four) proteins A−D interact with protein 1. Thus, 1 is labeled with a FRET donor, A−D are labeled with four different cassettes each having the same TBET-donor.
That donor also serves as a FRET acceptor for the dye on protein 1. Four different acceptor parts are used in the cassettes, ones that emit in distinct spectral regions. If 1 interacts with B and D simultaneously then two different emissions will be seen when the FRET donor is excited.
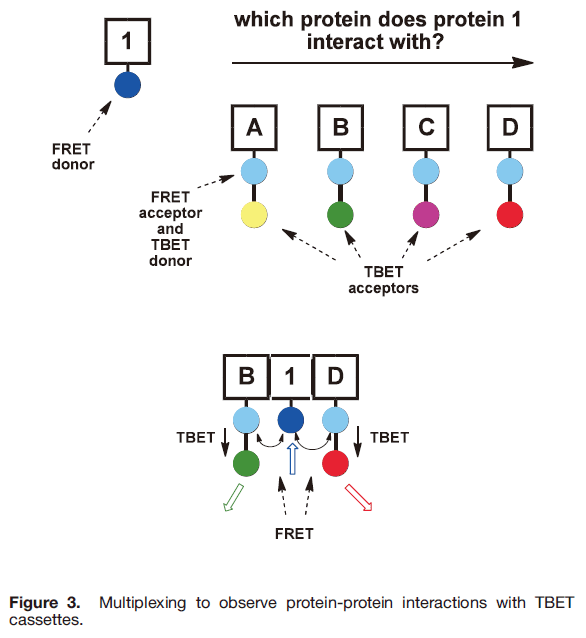
To obtain proof of principle, we engineered a system where in the protein Bovine Serum Albumin (BSA) was surface functionalized with biotin, and with a coumarin-based FRET-donor dye (Atto425). One of the cassettes was placed on avidin, the other was attached to streptavidin, and the FRET donor was excited in vitro . In that way it was shown that outputs from cassettes 4 and 5 could be observed simultaneously,
indicative of the type of interaction shown between the proteins B, 1, and D in Figure 3.
The next step was to attempt to observe this interaction in living cells. Figure 4 shows data obtained by using non-covalently bound peptide transporter systems like Pep-1 (Chariot) 35, 36) to import the dye-labeled proteins into cells. Excitation of the FRET donor in the presence of only 4-streptavidin gives emission at about 600 nm (though some leakage into the red channel is observed) (Figure 4a). When both 4- streptavidin + 5-avidin were introduced then fluorescence is seen in the 600 nm and 650 nm channels indicative of two FRET interactions exciting both the TBET cassettes simultaneously (Figure 4b).
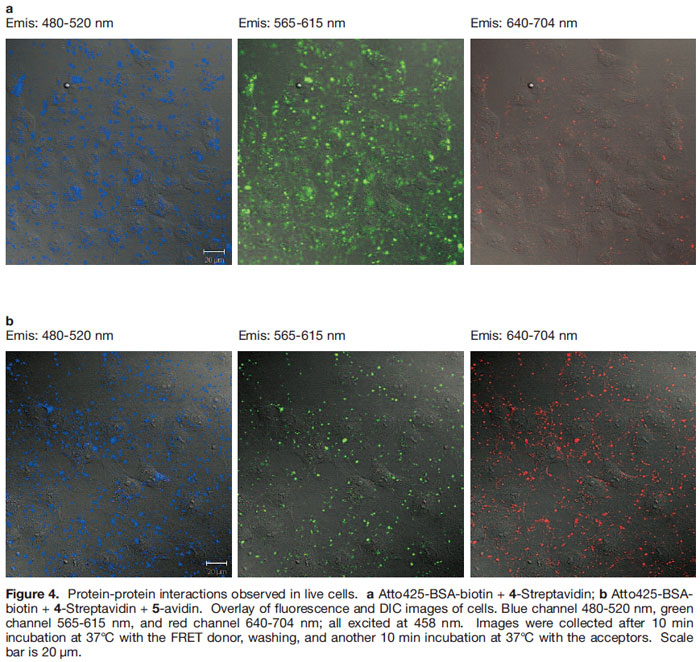
▲ページのトップへ
Application of TBET Cassettes In Measurement of Intracellular pH
Cassette 6 illustrates how TBET can be used to produce probes for intracellular pH(pHi) .37) In its protonated form, energy transfer from the xanthene donors to the BODIPY acceptor is efficient, so excitation at 488 nm leads to red fluorescence (ca 600 nm). At pH values above 6.5, however, the xanthate donor exists in the phenolate form, energy transfer is no longer efficient, and excitation at 488 nm gives green (fluorescein-like) fluorescence from the donor. Attributes of this system are: (i) it is always fluorescent, irrespective of the pH in the cell; (ii) the presence of two donors doubles the absorption maxima, ie the crosssection for collection of photons by the dye is high; and, (iii) the quantum yield of this system when excited is superior to many other pHi markers. 37) More recently, a Chinese group has reported the same concept but using an analog of cassette 3 with a coumarin donor and a simple rhodamine acceptor. 38)
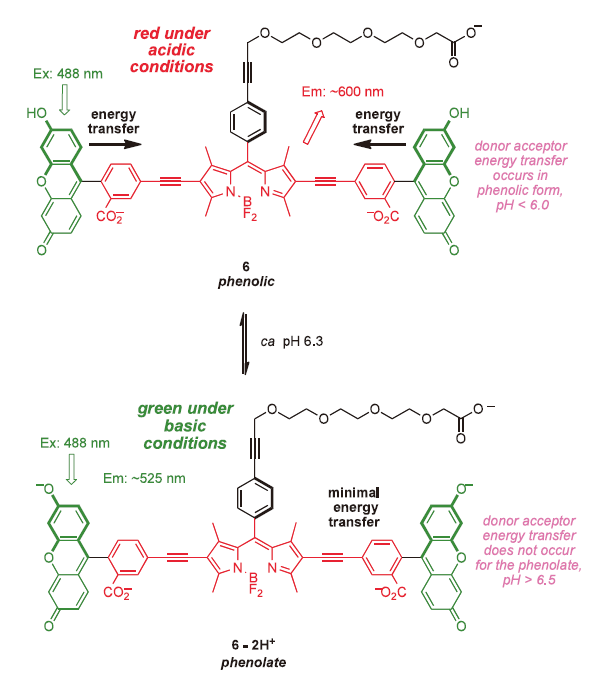
In our view, it is justifiable to introduce a distinction between stains and probes for gauging intracellular pH (pHi).37) When cells are bathed in a medium containing a pH-sensitive stain, this may permeate across the lipid bilayer and give a “global indication” of pHi. Conversely, when a fluorescent pH probe is attached to a protein or some other biomolecule, and that conjugate is then introduced into a cell (by whatever method) then the probe may be used to track the location of the biomolecule target in the cell and the pH of its immediate environment. Stains do not have to have a functional group for attachment to biomolecules, and they need not give brilliant fluorescence. Indeed, most stains could not be used as probes because they are insufficiently fluorescent to be detected at small intracellular concentrations with in the cell.
In 2008 we reported that when the non-covalently bound protein carrier Pep-1 (and other synthetic systems we designed) was used to introduce fluorescently labeled proteins into COS-7 cells at 37℃, they are imported into endosomes, but into the cytosol when the experiment is performed at 4℃. The second outcome is the desired one since to track the natural movement of proteins inside cells they cannot be trapped in endosomes.
Endosomes in cells are markedly more acidic (pH 5.0−5.5) than the cytosol,39) and they appear as vesicles when stained.
Thus, it follows from the discussion above that Pep-1 mediated import of a protein labeled with 6 at 37℃ should place the conjugate in endosomes where the probe would fluoresce red. However, if the import were performed at 4℃ then the probe would fluoresce green and red in the {neutral} cytosol. In the event, import of BSA-6 at 37℃ gave almost nothing in the green channel and red vesicles in the long wavelength one (Figure 5a), whereas import at 4℃ gave diffuse green and red fluorescence in the cytosol (Figure 5b; the intensity is weak relative to Figure 5a where the probe is concentrated in vesicles). This is exactly the data that was expected from these experiments.
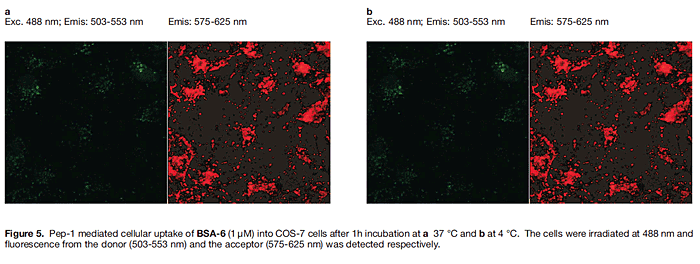
▲ページのトップへ
Outlook and Conclusions
There are more applications of TBET in biotechnology, some we cannot yet disclose, others that we are working on, and some based on published observations that have not yet been applied. For instance, cassettes 7 and 8 provide an answer to a question few researchers are asking at the moment: “how to obtain relatively long wavelength (>520 nm) chemiluminescence from probes based on luminol?” 20) Activation of these cassettes with peroxide in the presence of a metal salt causes energy transfer from the luminol part to the acceptors shown, hence the probes fluoresce green and red (corresponding to fluorescence from fluorescein and Nile Red, respectively) over a period of a second or two. This may be useful because methods to detect chemiluminesce can be more sensitive than fluorescence-based techniques.
In general, the notion of through-bond energy transfer, even if it is just one of several possible mechanisms of donor-acceptor energy transfer, is an exciting motivational force for the development of new fluorescent probes.
|